Shapeshifting Particles Yield Nobel Prize in Physics
Tuesday, October 6th, 2015October 6, 2015
Tuesday, October 6, two scientists were awarded the Nobel Prize in physics for their discovery that tiny subatomic particles called neutrinos have mass. Takaaki Kajita of Japan and Arthur B. McDonald of Canada shared the prize. The subject of their work, neutrinos, are so astonishingly small that they barely seem to exist at all. Thousands of billions of neutrinos constantly stream through Earth—and through the matter of our bodies—like wind through a screen door. Kajita and McDonald confirmed a remarkable property of the neutrino. As the particle moves through space, it can oscillate, or shift, between three different types. A mathematical consequence of this strange shapeshifting behavior is that at least one type of neutrino must have mass.
Scientists had previously doubted that neutrinos had any mass at all because they move so fast—nearly at the speed of light. In theory, only massless particles can travel at the speed of light. But unlike photons (particles of light), neutrinos barely interact with ordinary matter. Atoms, the building blocks of matter, can readily deflect or absorb photons, forcing light to travel in a zigzagging path. But neutrinos are so tiny, even compared to microscopic atoms, that they simply pass straight through the empty space between and within atoms. Only a handful among billions and billions of neutrinos ever bumps into an atom’s nucleus (core) or the electrons that surround it.
The three types of neutrinos are named after larger “partner” particles: (1) the electron, (2) the muon, and (3) the tau. Each type of neutrino is produced by a different physical process. For example, nuclear reactions in the sun’s core produce electron-neutrinos. High-energy particles called cosmic rays produce muon-neutrinos when they strike Earth’s atmosphere.
Despite the enormous challenge of detecting these ghostly particles, scientists have built machines that measure the rare collisions between neutrinos and bits of matter. Kajita worked at one such machine, the Super-Kamiokande detector, buried deep beneath Earth’s surface. In 1998, Kajita showed that far fewer muon-neutrinos from cosmic rays were showing up in the detector than the amount predicted by theory. In 2001, McDonald, working at another detector, the Sudbury Neutrino Observatory, showed that only a third of the neutrinos coming from the sun were electron-neutrinos—even though the sun only produces electron-neutrinos.
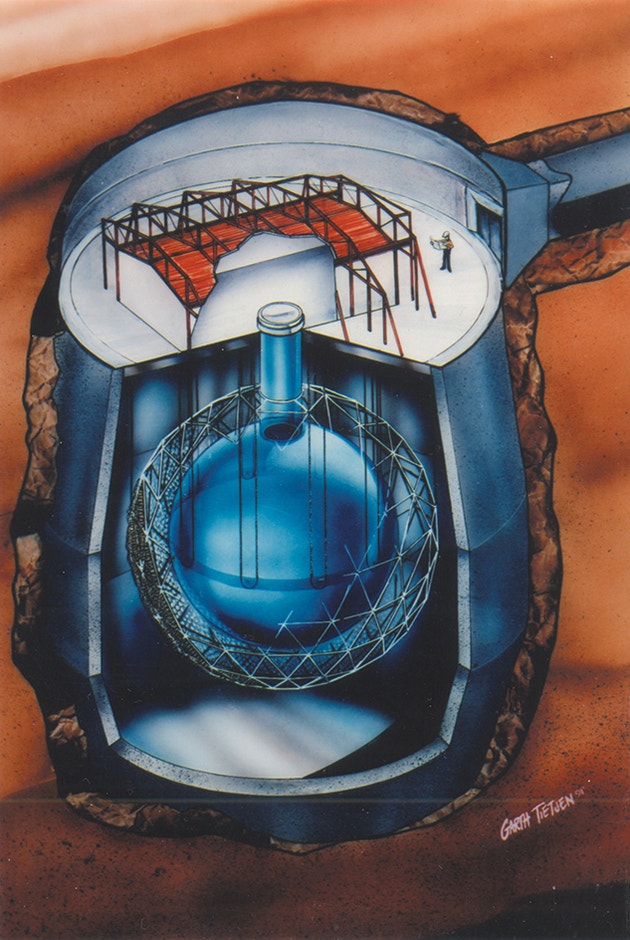
The Sudbury Neutrino Observatory consists of a large spherical water tank surrounded by sensors. The sensors detect flashes of light that occur when neutrinos interact with the water. (Sudbury Neutrino Observatory)
Kajita and McDonald’s detailed measurements provided conclusive evidence that the neutrinos from cosmic rays and the sun had not gone missing—they simply changed their identities on their way to the detectors. This shapeshifting ability—oscillation—is explained by a branch of physics called quantum mechanics. In quantum mechanics, a particle’s state—which includes its mass, energy, position, and speed—can be described mathematically as a “wave of probability.” In other words, a neutrino’s state, at any point in time, is not set in stone. Instead, the particle’s state is smeared out among a range of probabilities, which are distributed in a wavelike pattern. At certain points on a neutrino’s “wave of probability,” its state is most likely to be an electron-neutrino. At other points, its state is more likely to be a muon- or tau-neutrino. Thus, by the time an electron-neutrino created in the sun’s core reaches Earth, a different state may have bobbed up on the crest of its probability wave—rendering the particle invisible to electron-neutrino detectors.
If neutrinos had no mass, the complex math of quantum mechanics indicates that oscillation would be impossible to observe. Since Kajita and McDonald showed that neutrinos oscillate, the only logical conclusion is that at least one type of neutrino must have at least some mass. Scientists have yet to measure a neutrino’s mass precisely. But the discovery that at least one version of the particle’s mass is nonzero has many important implications for physics.